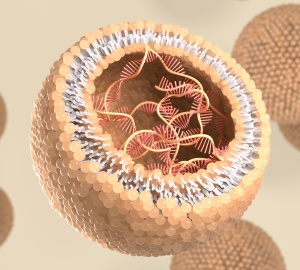
Lipid-based drug delivery (LBDD) systems, including liposomes, lipid nanoparticles (LNPs), solid lipid nanoparticles (SLNs), and nanostructured lipid carriers (NLCs), serve as customizable platforms designed for the precise delivery of pharmaceuticals and other therapeutic agents, such as vaccine components or oligonucleotides, to specific cells and tissues. LBDD effectively addresses challenges associated with conventional therapeutic formulations by enveloping bioactive molecules within a lipid vesicle. This encapsulation enhances bioavailability, distribution, and plasma half-life while simultaneously reducing systemic toxicity resulting from off-target activity. The adaptability of LBDD formulations is facilitated in part by the diverse range of lipids available for these applications. Through harnessing the biophysical characteristics of lipids, scientists can create a variety of LBDD systems capable of encapsulating and delivering an extensive array of biomolecules.
Structural Variances Among Phospholipids Employed in LBDD Systems
LBDD systems consist of lipid mixtures, with a particular focus on phospholipids (PLs) that comprise a hydrophilic head group and a hydrophobic tail. Among PLs, glycerophospholipids (GPLs) and sphingomyelin (SM), classified as a sphingolipid (SL), play crucial roles. GPLs consist of a polar head group and two fatty acyl tails connected to a glycerol backbone. Distinct polar head groups lead to the formation of various members, such as phosphatidylcholine (PC), phosphatidylethanolamine (PE), phosphatidylglycerol (PG), phosphatidylinositol (PI), phosphatidylserine (PS), and phosphatidic acid (PA). SM comprises a phosphocholine polar head group and a fatty acyl tail attached to a sphingosine backbone. The biophysical characteristics of these PLs are dictated by variations in their polar head groups and fatty acyl tails, each possessing unique properties that can be exploited in LBDD.
Biophysical Characteristics of PLs in LBDD Systems
The structural and operational attributes of an LBDD system are intricately linked to the physiochemical traits of its constituent phospholipids (PLs). PLs with diverse head group properties play a crucial role in facilitating the efficient delivery of encapsulated cargo to cells. The hydrophilic head of PLs plays a pivotal role in determining the particle's surface charge, which can be neutral, negative, or positive.1 At pH 7, PC, PE, and SM exhibit zwitterionic characteristics and an overall neutral charge. Neutral PLs are integral to membrane fusion within LBDD particles and can also be employed to modulate or adjust the net surface charge. Anionic PLs, such as PG, PI, PS, and PA, are commonly utilized for delivering small molecules, often incorporated into neutral LBDD systems to prevent aggregation during storage.
Synthetic cationic lipids, exemplified by DOTAP and DOTMA, are extensively utilized for delivering oligonucleotide cargoes due to their positively charged head groups, fostering electrostatic interactions with negatively charged oligonucleotides and cell membranes. While effective for mRNA, siRNA, or pDNA transfection in vitro, these lipids are associated with cytotoxicity.2 Ionizable cationic lipids like SM-102 and ALC-0315 exhibit a transient positive charge acquired at low pH, enabling the efficient encapsulation and delivery of anionic oligonucleotides without cytotoxic effects. These lipids have found application in formulating lipid nanoparticles (LNPs) for mRNA-based COVID-19 vaccines.3 Cayman now provides LNP Exploration Kits facilitating the preparation of LNPs containing SM-102 or ALC-0315 for oligonucleotide delivery.
PEGylated lipids, exemplified by ALC-0159 or DSPE-MPEG(2000), feature head groups that contribute distinctive functional properties to LBDD systems. The process of PEGylation enhances the circulation half-life of LBDD systems by conferring stealth properties upon them.4 This is achieved through PEGylated lipids inducing steric hindrance on the particle surface, diminishing protein adsorption, and preventing recognition and uptake by the mononuclear phagocyte system (MPS), a significant hurdle in LBDD system delivery. Furthermore, certain PEGylated lipids include terminal functional groups, such as amine or maleimide, enabling the conjugation of other molecules that enhance cellular targeting and uptake.
The fatty acyl tails of phospholipids (PLs) play a role in modulating membrane fluidity and permeability. PLs featuring short, unsaturated fatty acyl tails augment membrane fluidity.5 Double bonds, particularly cis-double bonds, introduce a 'kink' in the fatty acyl tail, expanding the volume occupied by the lipid and introducing lipid-packing defects, thereby increasing membrane permeability. To mitigate this effect, LBDD particles often incorporate cholesterol to fill packing defects and provide structural integrity.
Phase Organization of PLs in LBDD Systems
The diversity within LBDD systems stems from the amphiphilic nature of phospholipids (PLs) and their inclination to self-assemble into various phases in aqueous solutions. These phase assemblies are dynamic and offer opportunities to regulate encapsulation, membrane fusion, and cargo release. The configuration of lamellar or nonlamellar phase structures is influenced by the geometry of PLs, which is modulated by the degree of saturation in the fatty acyl tails.5,6
Fatty acyl tails featuring cis-double bonds occupy more space compared to saturated or trans-double bond-containing counterparts. PLs with similar-sized polar head groups and fatty acyl tails, such as PC, PG, PI, PS, and SM, adopt a cylindrical shape at neutral pH. These PLs tend to form the lamellar phase, characterized by neutral curvature, making them suitable for the creation of uni- or multi-lamellar liposomes. Nonlamellar phases, associated with cone- or inverted cone-shaped PLs, facilitate membrane fusion of LBDD systems with the target cell membrane.4,7
Cone-shaped PLs, like PA and PE, possess a small polar head group and bulky fatty acyl tails under physiological conditions, resulting in negative curvature. These lipids tend to organize into inverted phases, such as reverse micelles, capable of encapsulating hydrophilic molecules, or the hexagonal HII phase, featuring a highly ordered internal structure suitable for delivering large payloads.4-6,8 Lipids with a large polar head group and a single fatty acyl tail, such as lysophospholipids, exhibit an inverted cone shape, forming spherical micelles with positive curvature. Micelles can be employed for encapsulating poorly soluble, hydrophobic molecules.4
The phase transition of phospholipids (PLs) provides a strategic means to regulate cargo encapsulation and delivery, as each PL has a characteristic phase transition temperature (Tm), representing the temperature needed to induce a change in the lipid's physical state.6 The Tm of a PL is influenced by the length and saturation of its fatty acyl tails. In general, lipids with shorter, unsaturated fatty acyl tails exhibit lower Tms than those with longer, saturated fatty acyl tails. The lipid bilayer becomes more fluid at temperatures above the Tm, facilitating cargo encapsulation during preparation and the subsequent release of encapsulated cargo.
This property is exemplified in LBDD systems such as solid lipid nanoparticles (SLNs) and nanostructured lipid carriers (NLCs).9 Both SLNs and NLCs consist of lipids, including glycerolipids, fatty acids, and sterol lipids, and are stabilized by emulsifiers or surfactants. Notably, SLNs are comprised of solid lipids, while NLCs incorporate a mixture of solid and liquid lipids. The use of SLNs and NLCs enables both cargo retention during storage and prolonged cargo release in vivo.
Applications of LBDD Systems
LBDD systems represent a promising approach to mitigate the limitations of traditional therapeutic formulations. While lipid nanoparticles (LNPs) have gained recent attention as crucial components of mRNA-based COVID-19 vaccines, the utilization of lipids for delivering encapsulated agents has roots dating back to the 1960s.4 To date, 29 LBDD formulations have received approval for diverse applications, including cancer treatment, management of fungal and viral diseases, and use as analgesics or immunosuppressive agents.
The primary phospholipids (PLs) employed in LBDD systems are phosphatidylcholines (PCs), phosphatidylethanolamines (PEs), phosphatidylglycerols (PGs), and phosphatidylserines (PSs), particularly their saturated dimyristoyl (14:0/14:0), dipalmitoyl (16:0/16:0), distearoyl (18:0/18:0), and unsaturated dioleoyl (18:1/18:1) variants. Sphingomyelin (SM) has also been successfully incorporated into clinically approved LBDD formulations, as exemplified by Marqibo®, an FDA-approved form of vincristine, a chemotherapeutic agent, encapsulated in SM/cholesterol nanoliposomes.6 This demonstrates the versatility and efficacy of LBDD systems across various therapeutic domains.
Enhancing LBDD System Efficacy through Functional Modifications
In addition to the meticulous selection of component lipids, LBDD systems can be endowed with supplementary functional properties to further enhance their effectiveness. Targeted LBDD systems capitalize on receptor-ligand interactions to selectively target specific cell populations. Ligands, such as small molecules, peptides, or antibodies, can be affixed to the surface of lipid particles, forming a targeted LBDD system that binds to its corresponding receptor expressed by the target cell population. This targeted approach allows LBDD systems to be directed toward specific cells. For instance, liposomes modified with surface antibodies, known as immunoliposomes, have been employed to target doxorubicin specifically to tumor cells expressing ganglioside M3 (GM3).10 This exemplifies the customization of LBDD systems for precise and targeted therapeutic delivery.
Available from Cayman
Creating a successful LBDD system demands a comprehensive strategy that considers both the properties of the encapsulated cargo and the lipid constituents. The careful selection of high-quality lipids is paramount for the efficacy of your LBDD formulation. Cayman provides an extensive range of high-purity lipids suitable for the preparation of LBDD particles. Additionally, our contract services team is available for collaboration, offering custom synthesis of lipid components for LNPs to meet your specific requirements.
Original article written by Melissa A. Parsey, Ph.D, Cayman Chemical.
References:
1. Beltrán Gracia, E., López Camacho, A., Higuera Ciapara, I., et al. Nanomedicine review: Clinical developments in liposomal applications. Cancer Nanotechnol. 10, 11 (2019).
2. Zhi, D., Bai, Y., Yang, J., et al. A review on cationic lipids with different linkers for gene delivery. Adv. Colloid Interface Sci. 253, 117-140 (2018).
3. Schoenmaker, L., Witzigmann, D., Kulkarni, J.A., et al. mRNA-lipid nanoparticle COVID-19 vaccines: Structure and stability. Int. J. Pharm. 601, 120586 (2021).
4. Tenchov, R., Bird, R., Curtze, A.E., et al. Lipid nanoparticles─from liposomes to mRNA vaccine delivery, a landscape of research diversity and advancement. ACS Nano 15, 16982-17015 (2021).
5. Holthuis, J.C.M. and Menon, A.K. Lipid landscapes and pipelines in membrane homeostasis. Nature 510(7503), 48-57 (2014).
6. Li, J., Wang, X., Zhang, T., et al. A review on phospholipids and their main applications in drug delivery systems. Asian J. Pharm. Sci. 10(2), 81-98 (2015).
7. Cullis, P.R. and Hope, M.J. Lipid nanoparticle systems for enabling gene therapies. Mol. Ther. 25(7), 1467-1475 (2017).
8. Xu, Z., Seddon, J.M., Beales, P.A., et al. Breaking isolation to form new networks: pH-Triggered changes in connectivity inside lipid nanoparticles. J. Am. Chem. Soc. 143(40), 16556-16565 (2021).
9. Duong, V.-A., Nguyen, T.-T.L., and Maeng, H.-J. Preparation of solid lipid nanoparticles and nanostructured lipid carriers for drug delivery and the effects of preparation parameters of solvent injection method. Molecules 25(20), (2020).
10. Nam, S.M., Kim, H.S., Ahn, W.S., et al. Sterically stabilized anti-G(M3), anti-Le(x) immunoliposomes: Targeting to B16BL6, HRT-18 cancer cells. Oncol. Res. 11(1), 9-16 (1999).